Biochemistry in our Body
- Destin Hoffman
- Nov 29, 2022
- 8 min read
How does biochemistry play a part in our bodies' daily function? Read this article to understand more about this topic.
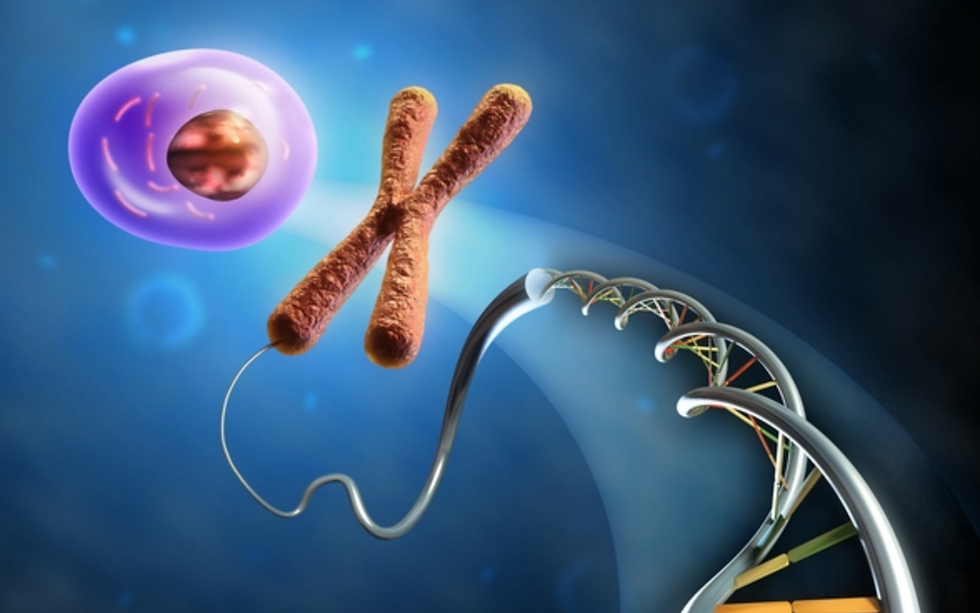
Introduction
Think back to what you ate for lunch. Did any of your lunch items have a “Nutrition Facts” label on the back of them? If so, and if you had a look at the food's protein, carbohydrate, or fat content, you may already be familiar with several types of large biological molecules. So what are these weird-sounding biological molecules? Well, they’re essentially the building blocks you need to maintain your body, just like nutrients. These building blocks are known as “biomolecules” or “biological macromolecules” because, on a molecular scale, they’re huge - made up of 10,000 or more atoms.
What do these biological macromolecules do?
There are four major classes of biological macromolecules (carbohydrates, lipids, proteins, and nucleic acids); each is an important cell component and performs a wide array of functions. Large biological molecules perform a wide range of jobs in an organism.
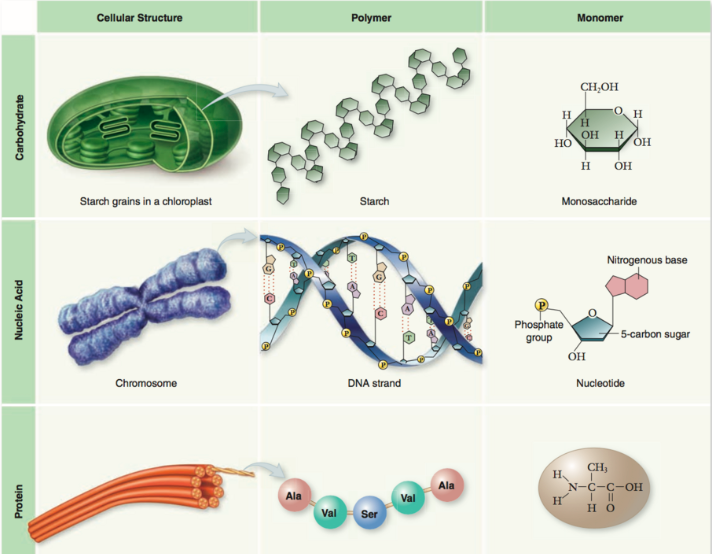
Some carbohydrates store the fuel for future energy needs, and some lipids are key structural components of cell membranes. Nucleic acids store and transfer hereditary information, much of which provides instructions for making proteins. Proteins themselves have perhaps the broadest range of functions: some provide structural support, but many are like little machines that carry out specific jobs in a cell, such as catalyzing metabolic reactions or receiving and transmitting signals. Below, we will be going over the structure and function of these four classes of large biomolecules.
Carbohydrates
Carbohydrates are macromolecules with which most consumers are somewhat familiar. To lose weight, some individuals adhere to “low-carb” diets. Athletes, in contrast, often “carb-load” before important competitions to ensure that they have sufficient energy to compete at a high level. Carbohydrates are, in fact, an essential part of our diet; grains, fruits, and vegetables are all natural sources of carbohydrates. Carbohydrates provide energy to the body, particularly through glucose, a simple sugar. Carbohydrates also have other important functions in humans, animals, and plants.
Carbohydrates can be represented by the formula (CH2O)n, where n is the number of carbon atoms in the molecule. In other words, the ratio of carbon to hydrogen to oxygen is 1:2:1 in carbohydrate molecules. Carbohydrates are classified into three subtypes: monosaccharides, disaccharides, and polysaccharides.
Monosaccharides (mono- = “one”; sacchar- = “sweet”) are simple sugars, the most common of which is glucose. In monosaccharides, the number of carbon atoms usually ranges from three to six. Most monosaccharide names end with the suffix -ose. Depending on the number of carbon atoms in the sugar, they may be known as trioses (three carbon atoms), pentoses (five carbon atoms), and hexoses (six carbon atoms).
The chemical formula for glucose is C6H12O6. In most living species, glucose is an important source of energy. During cellular respiration, energy is released from glucose, and that energy is used to help make adenosine triphosphate (ATP). Plants synthesize glucose using carbon dioxide and water through the process of photosynthesis, and the glucose, in turn, is used for the energy requirements of the plant. The excess synthesized glucose is often stored as starch that is broken down by other organisms that feed on plants.
Galactose (part of lactose, or milk sugar) and fructose (found in fruit) are other common monosaccharides. Although glucose, galactose, and fructose all have the same chemical formula (C6H12O6), they differ structurally and chemically (known as isomers) because of the different arrangements of atoms in the carbon chain (Figure 3).
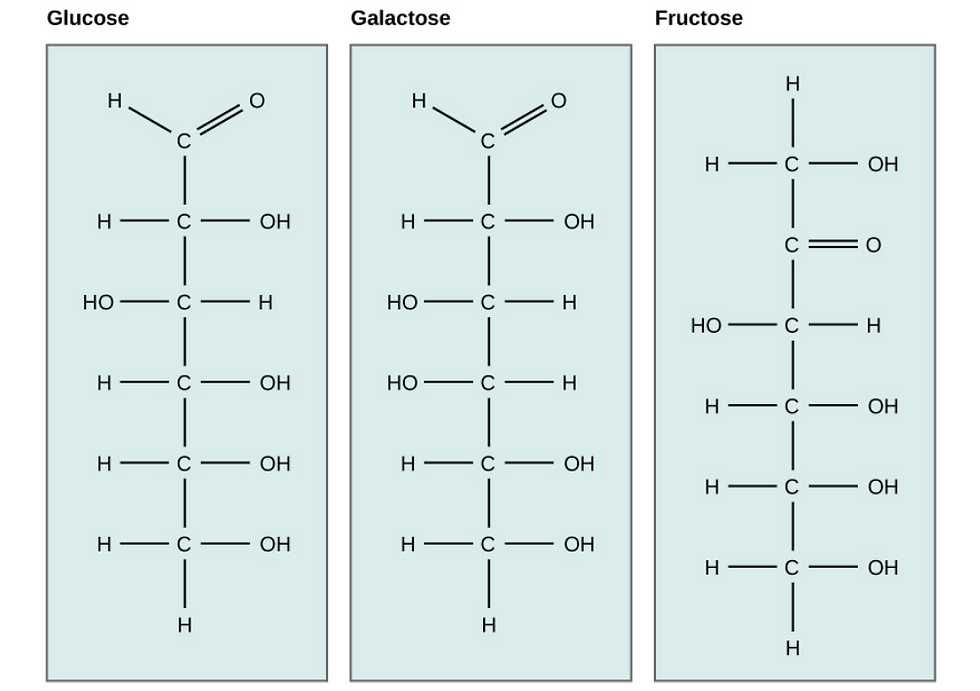
Figure 2: Glucose, galactose, and fructose are isomeric monosaccharides, meaning that they have the same chemical formula but slightly different structures.
Disaccharides (di- = “two”) form when two monosaccharides undergo a dehydration reaction (a reaction in which the removal of a water molecule occurs). Common disaccharides include lactose, maltose, and sucrose. Lactose is a disaccharide consisting of the monomers glucose and galactose. It is found naturally in milk. Maltose, or malt sugar, is a disaccharide formed from a dehydration reaction between two glucose molecules. The most common disaccharide is sucrose, or table sugar, which is composed of the monomers glucose and fructose.
Lipids
Lipids include a diverse group of compounds that are united by a common feature. Lipids are hydrophobic (“water-fearing”), or insoluble in water because they are nonpolar molecules. This is because they are hydrocarbons that include only nonpolar carbon-carbon or carbon-hydrogen bonds. Lipids perform many different functions in a cell. Cells store energy for long-term use in the form of lipids called fats. Lipids also provide insulation from the environment for plants and animals. For example, they help keep aquatic birds and mammals dry because of their water-repelling nature. Lipids are also the building blocks of many hormones and are an important constituent of the plasma membrane of our cells. Lipids include fats, oils, waxes, phospholipids, and steroids.
A fat molecule, such as a triglyceride, consists of two main components—glycerol and fatty acids. Glycerol is an organic compound with three carbon atoms, five hydrogen atoms, and three hydroxyl (–OH) groups. Fatty acids have a long chain of hydrocarbons to which an acidic carboxyl group is attached, hence the name “fatty acid.”. In a fat molecule, a fatty acid is attached to each of the three oxygen atoms in the –OH groups of the glycerol molecule with a covalent bond (Figure 3).
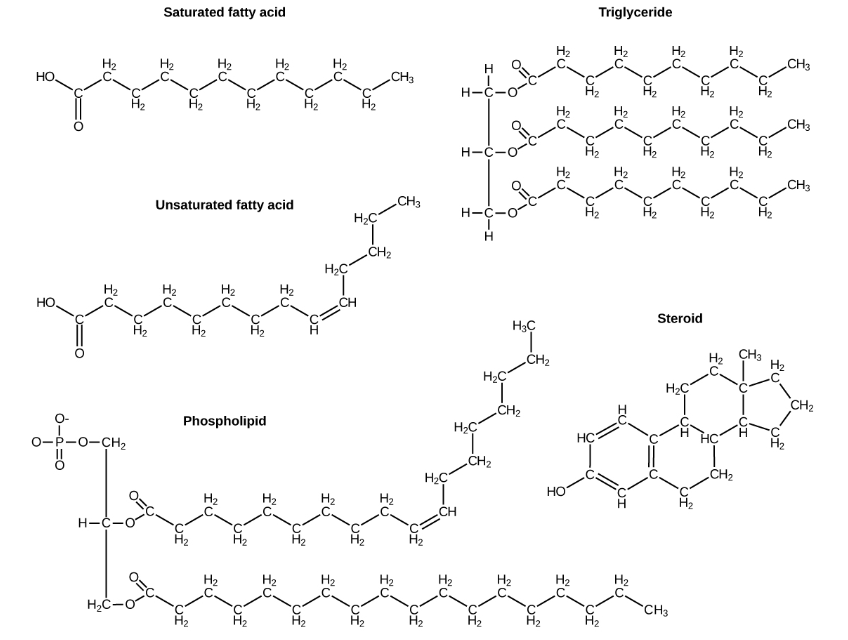
Figure 3: Lipids include fats, such as triglycerides, which are made up of fatty acids and glycerol, phospholipids, and steroids.
Fatty acids may be saturated or unsaturated. In a fatty acid chain, if there are only single bonds between neighbouring carbons in the hydrocarbon chain, the fatty acid is saturated. Saturated fatty acids are saturated with hydrogen; in other words, the number of hydrogen atoms attached to the carbon skeleton is maximized. When the hydrocarbon chain contains a double bond, the fatty acid is an unsaturated fatty acid.
Saturated fats tend to get packed tightly and are solid at room temperature. Animal fats with stearic acid and palmitic acid contained in meat, and fat with butyric acid contained in butter, are examples of saturated fats. Mammals store fats in specialized cells called adipocytes, where globules of fat occupy most of the cell. In plants, fat or oil is stored in seeds and is used as a source of energy during embryonic development.
Unsaturated fats or oils are usually of plant origin and contain unsaturated fatty acids. The double bond causes a bend or a “kink” that prevents the fatty acids from packing tightly, keeping them liquid at room temperature. Olive oil, corn oil, canola oil, and cod liver oil are examples of unsaturated fats. Unsaturated fats help to improve blood cholesterol levels, whereas saturated fats contribute to plaque formation in the arteries, which increases the risk of a heart attack. In short, saturated fats aren’t healthy in excess.
Proteins
Proteins are one of the most abundant organic molecules in living systems and have the most diverse range of functions of all macromolecules. Proteins may be structural, regulatory, contractile, or protective; they may serve in transport, storage, or membranes; or they may be toxins or enzymes. Each cell in a living system may contain thousands of different proteins, each with a unique function. Their structures, like their functions, vary greatly. They are all, however, polymers of amino acids, arranged in a linear sequence.
The functions of proteins are very diverse because there are 20 different chemically distinct amino acids that form long chains, and the amino acids can be in any order. For example, proteins can function as enzymes or hormones. Enzymes, which are produced by living cells, are catalysts in biochemical reactions (like digestion) and are usually proteins. Each enzyme is specific for the substrate (a reactant that binds to an enzyme) upon which it acts. Enzymes can function to break molecular bonds, rearrange bonds, or form new bonds. An example of an enzyme is salivary amylase, which breaks down amylose, a component of starch.
Hormones are chemical signalling molecules, usually, proteins or steroids, secreted by an endocrine gland or group of endocrine cells that act to control or regulate specific physiological processes, including growth, development, metabolism, and reproduction. For example, insulin is a protein hormone that maintains blood glucose levels.
Amino acids are the monomers that makeup proteins. Each amino acid has the same fundamental structure, which consists of a central carbon atom bonded to an amino group (–NH2), a carboxyl group (–COOH), and a hydrogen atom. Every amino acid also has another variable atom or group of atoms bonded to the central carbon atom known as the R group. The R group is the only difference in structure between the 20 amino acids; otherwise, the amino acids are identical (Figure 8).

Figure 4: Amino acids are made up of a central carbon bonded to an amino group (-NH2), a carboxyl group (-COOH), and a hydrogen atom. The central carbon's fourth bond varies among the different amino acids, as seen in these examples of alanine, valine, lysine, and aspartic acid.
The sequence and number of amino acids ultimately determine a protein’s shape, size, and function. Each amino acid is attached to another amino acid by a covalent bond, known as a peptide bond, which is formed by a dehydration reaction. The carboxyl group of one amino acid and the amino group of a second amino acid combine, releasing a water molecule. The resulting bond is the peptide bond.
As discussed earlier, the shape of a protein is critical to its function. Proteins have four levels of structure: primary, secondary, tertiary, and quaternary (Figure 5)
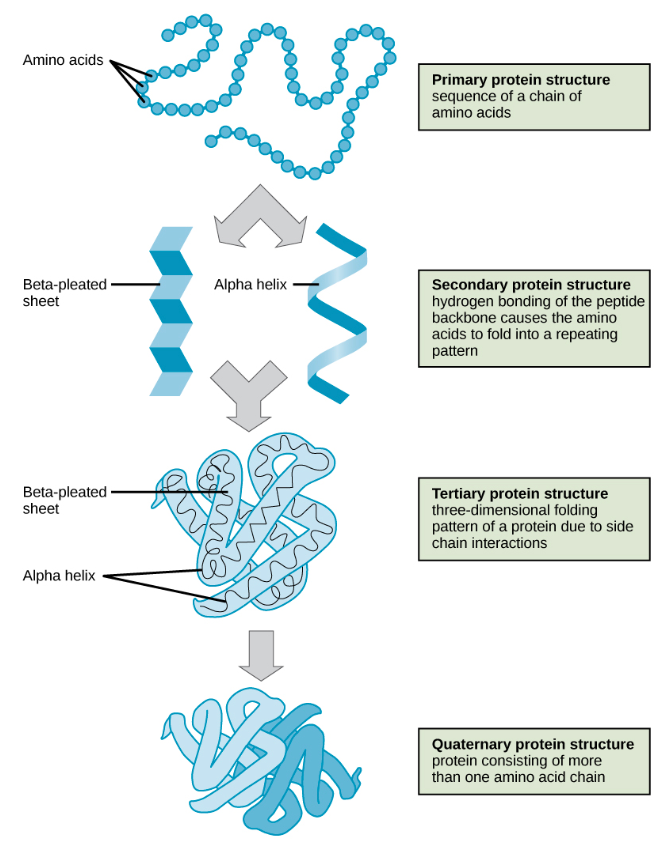
Figure 5: The four levels of protein structure can be observed in these illustrations.
Each protein has its own unique sequence and shape held together by chemical interactions. If the protein is subject to changes in temperature, pH, or exposure to chemicals, the protein structure may change, losing its shape in what is known as denaturation. The denaturing and misfolding of vital proteins in our body are believed to be the primary cause of Alzheimer's disease, Parkinson's disease, Huntington's disease, Creutzfeldt-Jakob disease, cystic fibrosis, Gaucher's disease and many other degenerative and neurodegenerative disorders.
Nucleic Acids
Nucleic acids are key macromolecules in the continuity of life. They carry the genetic blueprint of a cell and carry instructions for the functioning of the cell.
The two main types of nucleic acids are deoxyribonucleic acid (DNA) and ribonucleic acid (RNA). DNA is the genetic material found in all living organisms, ranging from single-celled bacteria to multicellular mammals.
The other type of nucleic acid, RNA, is mostly involved in protein synthesis. The DNA molecules never leave the nucleus, but instead, use an RNA intermediary to communicate with the rest of the cell. Other types of RNA are also involved in protein synthesis and its regulation.
DNA and RNA are made up of monomers known as nucleotides. The nucleotides combine with each other to form a polynucleotide, DNA or RNA. Each nucleotide is made up of three components: a nitrogenous base, a pentose (five-carbon) sugar, and a phosphate group (Figure 6). Each nitrogenous base in a nucleotide is attached to a sugar molecule, which is attached to a phosphate group.
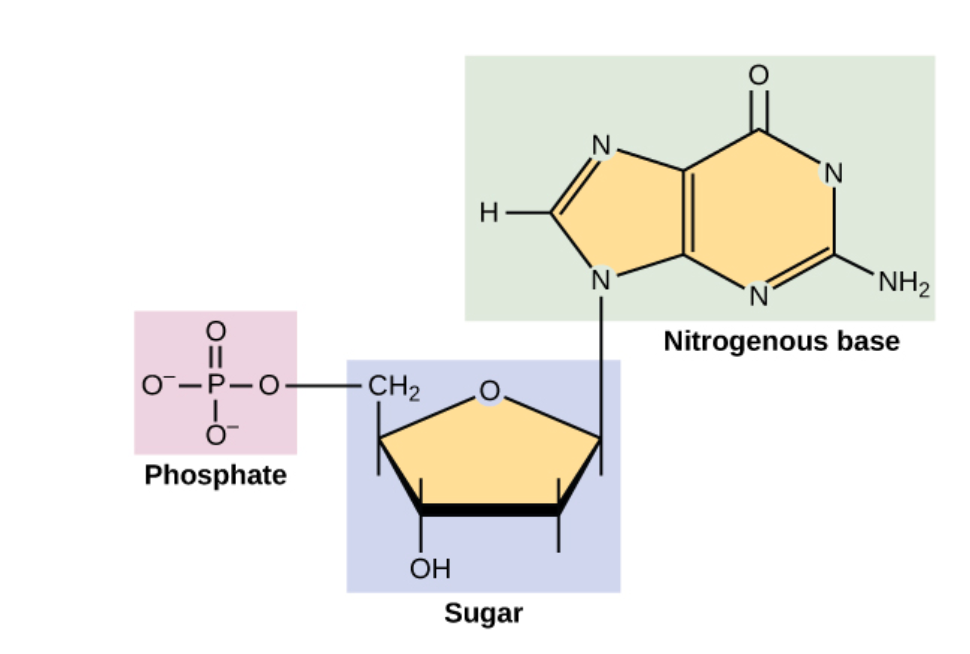
Figure 6: A nucleotide is made up of three components: a nitrogenous base, a pentose sugar, and a phosphate group.
Conclusion
Living things are carbon-based because carbon plays such a prominent role in the chemistry of living things. The four covalent bonding positions of the carbon atom can give rise to a wide diversity of compounds with many functions, accounting for the importance of carbon in living things. Carbon, Hydrogen, Nitrogen, Oxygen Phosphorus, and Sulfur make up the macromolecules of our body, and thus, it can be said that CHNOPS are the common elements that make up all living organisms on Earth.
The impact of nutrition on both physical and mental health has gained much interest in recent years. Emerging studies are finding more strong connections between diet, gut health and the microbiome, and various diseases (both physical and mental). Part of the research into these connections is being undertaken by the field of nutritional biochemistry which investigates the mechanisms which underly these interactions between diet and disease. If you’re interested in nutrition, I recommend looking into the exciting field of nutrigional biochemical research.
Citations:
[1] Scheinhart, Wolfgang. “Is There More to Your Body than Biochemistry?” Https://Www.juneva.com/, www.google.com/url?q=www.juneva.com/2022/11/05/is-there-more-to-your-body-than-biochemistry/&sa=D&source=docs&ust=1669733218914144&usg=AOvVaw00MA1WdjeNmxLlHV1Ekhhi. Accessed 29 Nov. 2022.
[2] Malmquist, Sarah, and Kristina Prescott. “4.1 Biological Molecules.” Open.lib.umn.edu, open.lib.umn.edu/humanbiology/chapter/4-1-biological-molecules/#:~:text=There%20are%20four%20major%20classes.
[3] Chaudhuri, Tapan K., and Subhankar Paul. “Protein-Misfolding Diseases and Chaperone-Based Therapeutic Approaches.” FEBS Journal, vol. 273, no. 7, Apr. 2006, pp. 1331–1349, 10.1111/j.1742-4658.2006.05181.x.
[4] “What Is Nutritional Biochemistry?” News-Medical.net, 14 May 2021, www.news-medical.net/life-sciences/What-is-Nutritional-Biochemistry.aspx.
[5] Neustadt, Dr John. “Change Your Biochemistry to Change Your Health.” NBI, 27 Feb. 2018, www.nbihealth.com/change-biochemistry-change-health/#:~:text=Biochemistry%20is%20the%20process%20by. Accessed 29 Nov. 2022.
Comments